Rhône AGG Description/Documentation
The experimental setup is described in this section.
Experiment Description
Time frame
The model forcing consists of 4 years: starting
August 1, 1985, and ending July 31, 1989.
The first year (ending July 31, 1986)
is to be treated as a spin-up year,
so we ask that the participants provide results
for the final three years (August 1, 1986-July 31 1989).
The simulations begin in late summer due to the large snow
component of the Rhône hydrological cycle.
The simulations extend into the summer of
1989 in order to encompass the principal
snow melt season as it has a significant
impact on the discharge at the gauging stations
within the Alps.
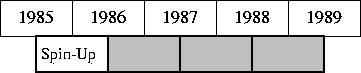
FIG. 3.1 The distribution contains
forcing from August 1, 1985, through July 31, 1989 (lower bar).
The first 12 month period is to be used as model
spin-up, while the remaining three years (shaded region)
are to be reported.
Temporal Interpolation and Spin Up
The atmospheric forcing data are to be linearly interpolated from
the three hour time step down to the model time step, except for the solid
and liquid precipitation
rates, which are to be held constant over the three-hour time interval.
We request that modelers use time steps in their models of no
more than three hours (we assume that the majority will run with
time steps considerably smaller than this).
The first forcing values correspond to the initial state
(there are 365 x 8 + 1 time steps for the first year:
1987-1988 is a leap year so there are 366 x 8 time periods),
all other values correspond to the end of the current three-hour
time step.
Snow cover and soil ice should be initialized at zero at the
start of the spin-up year (August 1, 1985-July 31, 1986).
The models are then requested to
``spin-up'' repeatedly using the first 365 days of the provided
data. It is possible that snow will remain throughout the
year in some models at some of the higher elevation points.
Once a reasonable equilibrium state
has been achieved, we ask that the modelers simulate the
remaining three years. We ask that the groups only report
results for the last three years.
Experiment 1: The Entire Domain
The first experiment consists of running the SVAT scheme
for the 1471 grid points for at least 1 year of spin-up, and then
for the remaining three years which are to be reported.
We ask that all modelers use the provided forcing
(Table 3.9)
and the mandatory parameter
datasets (shown in
Table 3.1):
other parameters
either included in the distribution (such as rooting depth,
wilting point water content, etc.) or not listed are up
to the discretion of the modelers. The effective parameters
on the distribution may be used, or the modelers can calculate
their own effective parameters using the correspondence data.
Model results will be used to calculate the river discharge
with the MODCOU model at CNRM. The results will be compared to the daily
observed stream-flow at various gauging stations. The modeled snowpack depth
will also be compared with the observations, and the various
model results will be inter-compared. This simulation will serve as the high
resolution baseline result.
Experiment 2: Aggregated grid boxes
The second experiment consists of three simulations
at two different grid resolutions.
In the first test (Experiment 2a),
each SVAT scheme is to be run over the same
4-year period for 20 sub-areas of the Rhône basin.
The boundaries for each of these areas is determined using
a 1 x 1 degree grid (which is consistent with the grid
configuration used by the GSWP). The approximate divisions
are shown in
Fig. 3.1,
and the actual mask is
shown on the left panel of
Fig. 3.2.
We provide the area-average forcing and aggregated parameters
for each of these sub-areas.
The surface parameters have
been aggregated for the 1 x 1 degree grid
using the basic rules which are outlined later in this chapter together with
the provided 8 x 8 km effective parameters (as opposed to the correspondence
tables). The provided effective soil hydraulic parameters for the 1 x 1 degree grid
are calculated using the grid
box average soil texture, however, modelers may use other methods for
aggregation (such as directly aggregating the 8 x 8 gridded
soil hydraulic parameters, etc.).
In addition, we have provided the mask for the sub-areas
so that modelers may either use the default aggregated parameters,
or they may aggregate the vegetation parameters and soil texture
as they see fit. They may use the masks to define
statistical distributions of topography, soils, etc., or to
define sub-grid mosaics or tiles.
We ask that participants running tile-type schemes
only report the basin average output variables.
The purpose of this experiment is to see the impact of changing the spatial scale
from 8x8 km to that of a relatively high resolution climate model (and the same
scale used in GSWP) on the simulations of the various hydrological components.
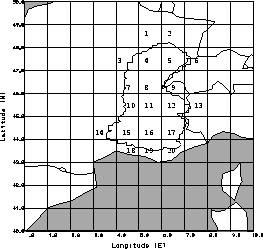
FIG. 3.2 The Rhône basin model domain (outlined area) together
with the GSWP grid. The 20 boxes for Experiment 2
are indicated.
The second test (Experiment 2b) consists of
running the scheme in the same manner as in Experiment 2a, but
for 60 approximately 1/2 x 1/2 degree grid boxes.
As in Experiment 2a, aggregated forcing will be provided, and modelers
may use either the provided aggregated parameters or their own
set of aggregated parameters (based on their 8 x 8 km values).
The purpose of this experiment is to examine an
intermediate spatial scale in order to give a basic idea
of whether the impact of scaling is linear, nonlinear, etc.
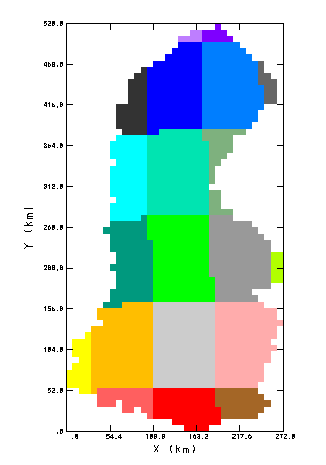
FIG. 3.3a The Rhône basin model domain grid mask
for Experiment 2a using a 1.0 degree grid.
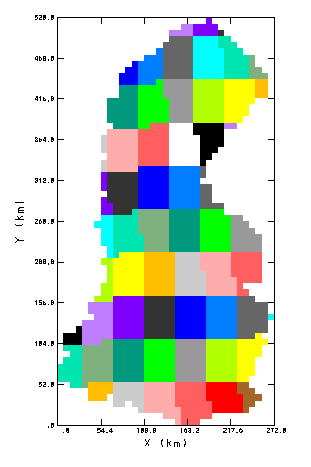
FIG. 3.3b The Rhône basin model domain grid mask
for Experiment 2b using a 0.5 degree grid.
A third test (Experiment 2c) consists of
running the scheme in the same manner and grid configuration
as in Experiment 2a,
but using the dominant parameters only. We will provide
the dominant parameters for this experiment, and the forcing
from Experiment 2a will be used. Mosaic type schemes are
to run this simulation with one tile for each grid box
(using the dominant parameters).
The goal of this experiment is to examine the impact of using
effective verses dominant parameters.
Experiment 3: Aggregated Basins
The aggregated forcing
(including the fractional coverage of liquid and solid precipitation
at each three hour time step)
and surface/soil parameters are
provided
for three sub-catchments within the Rhône basin, so that
this experiment consists in running your SVAT model for
3 separate stand-alone or point simulations. The sub-basin
masks are shown in
Fig. 3.4:
the sub-basins are
for the Ardeche, Saone and Durance rivers.
The main interests of this experiment are that the basin-scale
simulated discharge can be directly compared to the observations,
and the basins comprise three distinct climate regimes:
the Ardeche has relatively light snowfall and heavy convective
rainfall in the summer, the Saone is representative of a continental
climate, and the Durance is a relatively high altitude basin (the mean
altitude is approximately 2000 m) which
receives considerable snowfall.
As in Experiment 2, we provide the mask for the three basins so
that modelers may either use the default aggregated parameters,
or they may aggregate the vegetation parameters and soil texture
as they see fit. As in Experiment 2,
we ask that participants running tile-type schemes
report only the basin average output variables.
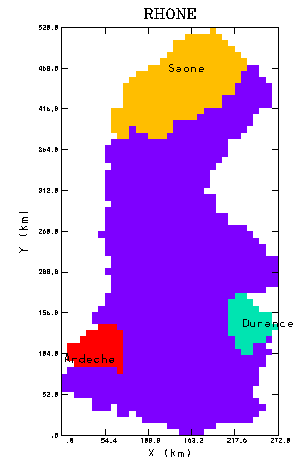
FIG. 3.4 The three sub-basins within the Rhône basin model domain
used for Experiment 3: the Ardeche, the Durance and the Saone.
See Table 3.1 for basin characteristics.
TABLE 3.1 Experiment 3 basin
characteristics (shown in Fig. 3.4).
The contributing area and average grid box altitudes are shown.
Basin |
Area (km2) |
Altitude (m) |
Ardeche | 2240 | 677 |
Durance | 2170 | 2149 |
Saone | 11700 | 330 |
Calibration
The stream flow data will be provided for two relatively small
contrasting catchments within the Rhône basin over the
four-year simulation period.
Two masks are provided on the distribution
so that modelers can extract the needed parameters and
forcing from the 8 x 8 km grid data. In addition, a file containing
the contributing area for each of the 8 x 8 km grid boxes for the two basins
is provided so that modelers can
calculate the total runoff corresponding to the calibration basins.
The observed discharge has units of m3 s-1, so
that modelers will need to convert their total runoff using
the contributing area fractions. The model simulated
total runoff (surface and sub-surface or drainage) for a given time
period can be
evaluated using this data without the use of the routing model.
Please note: the contributing area fraction is only to be
used for calibration, it is not to be used in the
computation of the model runoff data which is to be reported as output
(to us).
The calibrated hydrological parameters can then be extrapolated
over the entire domain however the modelers prefer for
Experiment 1,
and they can be aggregated as modelers wish for
Experiments 2 and 3.
However, we ask that the parameters in
Table 3.1
not be changed from the provided values. Please note that
calibration is not mandatory, and that by default the results are
included in
Experiment 1,
output (we request no additional calibration output).
We would like each participant group to provide us with a brief
description as to which/what parameters were calibrated, how they
were extrapolated over the domain, and how they were aggregated for
Experiments 2 and 3.
Evaluation
The simulated gridded total daily surface runoff
and baseflow/drainage (Qs and Qsb, respectively: see
Chapter 5)
will be collected and used at CNRM as
inputs into the distributed hydrological
model MODCOU. The simulated runoff will then be compared directly with
observations. We initially plan on using several standard
metrics: the correlation (squared), the efficiency or Nash coefficient
(Nash and Sutcliffe, 1970),
the Root Mean Square error (RMS) and the ratio of the
simulated to the observed discharge. We will also compare the simulated grid-box
daily average snow depths with the observed values.
The discharge obtained using the aggregated
forcing and parameters will be compared to the observations
(Experiment 3, and
Experiment 2 for entire basin) and
to the discharge simulated in
Experiment 1. Other standard outputs
(such as surface fluxes, soil moisture, SWE, etc.) will also
be compared with those from
Experiment 1.
Initialization
The models are to assume that initially there is
no snow cover and no soil ice (on August 1). Note that some higher altitude
points may retain snow cover throughout the year.
Because the simulations begin in mid-to-late summer,
we recommend models initialize their root-zone soil moisture stores
using the following relationship:
|
(3.9)
|
where the left-hand-side of Eq. 3.9 represents the average volumetric
water content (m3 m-3) from the surface down to the base of
the root-zone soil layer.
This corresponds to SWI/2, where
the Soil Wetness Index is defined as
|
(3.10)
|
It is recommended that sub-root zone layers be initialized
using a water content w in the range
wwilt <= w <= wfc.
The soil temperature profile can be initialized
using the average annual air temperature as a first guess.
|